The Nature of the Soil
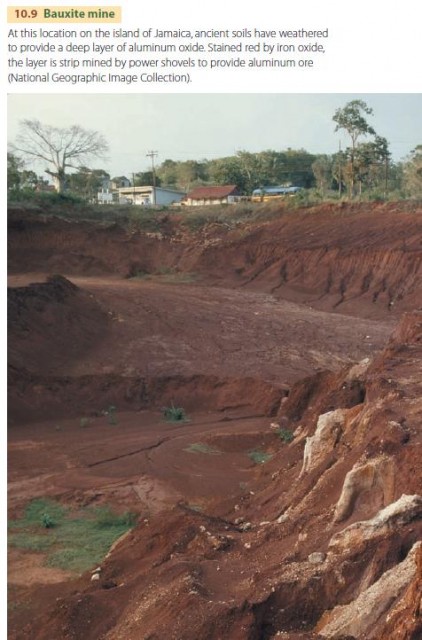
Vegetation is an important factor in determining soil qualities. For example, some of America's richest soils developed in the Middle West under a cover of thick grass (Figure 10.2). The deep roots of the grass, in a cycle of growth and decay, deposited nutrients and organic matter throughout the thick soil layer. In the Northeast, conifer forests provided a surface layer of decaying needles that kept the soil quite acid. This acidity allowed nutrients to be washed below root depth, out of the reach of plants. When farmed today, these soils need applications of lime to reduce their acidity and enhance their fertility.
Climate, measured by precipitation and temperature, is also an important determinant of soil properties. Precipitation controls the downward movement of nutrients and other chemical compounds in soils. If precipitation is abundant, water tends to wash soluble compounds, including nutrients, deeper into the soil and out of reach of plant roots.
Temperature acts to control the rate of decay of organic matter that falls to the soil from the plant cover or that is provided to the soil by the death of roots. When conditions are warm and moist, decay organisms work efficiently, consuming organic matter readily.
Thus, organic matter and nutrients in soils of the tropical and equatorial zones are generally low. Where conditions are cooler, decay proceeds more slowly, and organic matter is more abundant in the soil. Of course, if the climate is very dry or desert-like, then vegetation growth is slow or absent. No matter what desert temperatures are like, organic matter will be low.
Time is also an important factor. The characteristics and properties of soils require time for development. For example, a fresh deposit of mineral matter, like the clean, sorted sand of a dune, may require hundreds to thousands of years to acquire the structure and properties of a sandy soil.
Lastly, soil development is dependent on the parent material—the mineral matter that is available to make up the soil. Because different minerals weather at different rates to form different products, the starting material can be quite important.
Geographers are keenly interested in the differences in soils from place to place over the globe. The ability of the soils and climate of a region to produce food largely determines the size of the population it will support. In spite of the growth of cities, most of the world's inhabitants still live close to the soil that furnishes their food. And many of those same inhabitants die prematurely when the soil does not furnish enough food for all.
INTRODUCING THE SOIL
Soil includes matter in all three states—solid, liquid, and gas. Because the matter in these states is constantly changing and interacting through chemical and physical processes, soil is a very dynamic layer.
Soil includes mineral matter from rock material and organic matter from both live and dead plants and microorganisms. Soil scientists use the term humus to describe finely divided, partially decomposed organic matter in soils. Some humus rests on the soil surface, and some is mixed through the soil, having been carried down through lower soil layers by rainfall. Humus particles can make soil look brown or black.
We also find air and water in soil. Water contains high levels of dissolved substances, such as nutrients. Air in soils can have high levels of carbon dioxide or methane and low levels of oxygen.
Soil characteristics develop over a long period of time through a combination of many processes acting together. Physical processes break down rock fragments of regolith into smaller and smaller pieces.
Chemical processes alter the mineral composition of the original rock, producing new minerals. Taken together, these physical and chemical processes are referred to as weathering.
In most soils, the inorganic material is made of fine particles of mineral matter that are derived from the parent material (Figure 10.3). Over time, weathering processes soften, disintegrate, and break bedrock apart, forming a layer of regolith. Other kinds of regolith are mineral particles transported by streams, glaciers, waves, and water currents, or winds. For example, dunes formed of sand transported by wind are a type of regolith on which soil can form.
Although we may think that soil is found all over the world, large expanses of continents don't possess soil. For example, dunes of moving sand, bare rock surfaces of deserts and high mountains, and surfaces of fresh lava near active volcanoes do not have a soil layer.
SOIL COLOR AND TEXTURE
Color is the most obvious feature of a soil. Some color relationships are quite simple. For example, Midwest prairie soils are black or dark brown in color because they contain many humus particles. And the red or yellow soils of the Southeast are created by the presence of iron-containing oxides.
In some areas, soil color is inherited from the mineral parent material, but, more generally, the color is generated during soil formation. For example, dry climates often have soils with a white surface layer of mineral salts that have been brought upward by evaporation. In the cold, moist climate of the boreal forest, a pale, ash-gray layer near the top of the soil is created when organic matter and colored minerals are washed downward, leaving only pure, light-colored mineral matter behind.
The mineral matter of the soil consists of individual mineral particles that vary widely in size. The term soil texture refers to the proportion of particles that fall into each of three size grades—sand, silt, and clay (Figure 10.5). The finest soil particles, which are included in the clay size grade, are called colloids.
The coarsest particles—gravel—are ignored in discussing soil texture because they don't play an important role in soil processes.
A loam is a soil mixture containing a substantial proportion of each of the three grades. Loams are classified as sandy, silty, or clay-rich, depending on which grade is dominant (Figure 10.6). Why is soil texture important? Soil texture determines the ability of the soil to hold water. Coarse- textured (sandy) soils have many small passages between touching mineral grains that quickly conduct water through to deeper layers. But soils of fine particles have far smaller passages and spaces, so water will penetrate down more slowly and will tend to be held in the upper layers. We will return to the water-holding ability of soils later.
SOIL COLLOIDS
Soil colloids are particles smaller than one tenthousandth of a millimeter (0.0001 mm, 0.000,004 in.). Like other soil particles, some colloids are mineral, whereas others are organic. Mineral colloids are usually very fine clay particles. They have a thin, plate-like shape that reflect their layered chemical crystal structure. When these particles are well mixed in water, they remain suspended indefinitely, turning the water murky. Organic colloids are tiny bits of organic matter that are resistant to decay.
Soil colloids are important because their surfaces attract soil nutrients, dissolved in soil water. The nutrients are in the form of ions, and Figure 10.7 shows how they interact with a colloidal particle. Among the many ions in soil water, one important group consists of bases, which are ions of four elements: calcium (Ca + + ), magnesium (Mg + + ), potassium (K + ), and sodium (Na + ).
Bases are needed for plant growth. Colloids hold these ions, but also give them up to plants when they are in close contact with root membranes. Without soil colloids, most vital nutrients would be carried out of the soil by percolating water and taken away in streams, eventually reaching the sea.
SOIL ACIDITY AND ALKALINITY
Soil solution also contains the acid ions hydrogen (H + ) and aluminum (Al + + + ). Unlike the bases, they are not plant nutrients, and they tend to make soil solutions acidic overall. Acid ions have the power to replace the nutrient bases clinging to the surfaces of the soil colloids. As the acid ions displace the bases and build up, the bases are released to the soil solution. The bases are then gradually washed downward below rooting level, reducing soil fertility. When this happens, the soil acidity is increased.
Soils in cold, humid climates typically have high acidities, while in arid climates, soils are typically alkaline. Acidity can be corrected by applying lime, a compound of calcium, carbon, and oxygen (CaCO3), which removes acid ions and replaces them with the base calcium.
SOIL STRUCTURE
Soil structure refers to the way in which soil grains are clumped together into larger masses, called peds. The particles are bound together by soil colloids to create peds ranging from small grains to large blocks. Peds form when colloid-rich clays shrink as they dry out. Cracks form in the soil, which define the surfaces of the peds. Small peds, roughly shaped like spheres, give the soil a granular or crumb structure.
Larger peds provide an angular, blocky structure. Soils with a well-developed granular or blocky structure are easy to cultivate—a factor that's especially important for farmers using primitive plows that are drawn by animals. Soils with a high clay content can lack peds. They are sticky and heavy when wet and are difficult to cultivate. When dry, they become too hard to be worked.
SOIL MINERALS
There are two classes of soil minerals: primary and secondary. Primary minerals are compounds found in unaltered rock. These are mostly silicon- and oxygencontaining minerals with varying proportions of aluminum, calcium, sodium, iron, and magnesium. Primary minerals make up a large fraction of the solid matter of many kinds of soils, but they don't play an important role in sustaining plant or animal life.
When primary minerals are exposed to air and water at or near the Earth's surface, their chemical composition is slowly altered. Mineral alteration is a chemical weathering process that is explained in more detail in Chapter 8. The primary minerals are altered into secondary minerals, which are essential to soil development and to soil fertility.
The most important secondary minerals for soils are clay minerals, which hold base ions. They make up the majority of fine mineral particles. Some clay minerals hold bases tightly, and others loosely.
The clay minerals in a soil determine the soil's base status. If the clay minerals can hold abundant base ions, the soil is of high base status and will be highly fertile. If the clay minerals hold a smaller supply of bases, the soil is of low base status and is less fertile. Humus colloids have a high capacity to hold bases so they are associated with high soil fertility.
Mineral oxides are also significant secondary minerals. We find them in many kinds of soils, particularly those that have developed in warm, moist climates over very long periods of time (hundreds of thousands of years). Under these conditions, minerals are chemically broken down into simple oxides—compounds in which a single element is combined with oxygen.
Oxides of aluminum and iron are the most important oxides in soils. Two atoms of aluminum are combined with three atoms of oxygen to form the sesquioxide of aluminum (Al2O3). (The prefix sesqui means “one and a half” and refers to the chemical composition of one and one-half atoms of oxygen for every atom of aluminum.) In soils, aluminum sesquioxide and water molecules bind together to make the mineral bauxite. Where bauxite layers are thick and uniform, they are sometimes strip-mined as aluminum ore (Figure 10.9).
Iron sesquioxide (Fe2O3) combines with water molecules to produce limonite, a yellowish to reddish mineral that makes soils and rocks reddish to chocolate-brown. At one time, shallow accumulations of limonite were mined for iron.
SOIL MOISTURE
The soil layer is also a reservoir of moisture for plants. Soil moisture is a key factor in determining how the soils of a region support vegetation and crops.
The soil receives water from rain and from melting snow. Where does this water go? Some of the water simply runs off, flowing into brooks, streams, and rivers, and eventually reaching the sea. But some sinks into the soil. Of that, a portion is returned to the atmosphere as water vapor when soil water evaporates and when plant transpiration lifts soil water from roots to leaves, where it evaporates. These two processes are together called evapotranspiration. Some water can also flow completely through the soil layer to recharge supplies of ground water at depths below the reach of plant roots.
Suppose now that no further water enters the soil for a time. Excess soil water continues to drain downward, but some water clings to the soil particles. This water resists the pull of gravity because of the force of capillary tension. To understand this force, think about a droplet of condensation that has formed on the cold surface of a glass of ice water. The water droplet seems to be enclosed in a “skin” of surface molecules, drawing the droplet together into a rounded shape. That “skin” is produced by capillary tension, which keeps the drop clinging to the side of the glass indefinitely, defying the force of gravity. Similarly, tiny films of water stick to soil grains, particularly at the points of grain contacts. They remain until they evaporate or are absorbed by plant rootlets.
When a soil has first been saturated by water and then allowed to drain under gravity until no more water moves downward, the soil is said to be holding its storage capacity of water. For most soils, drainage takes no more than two or three days. Most excess water is drained out within one day.
Storage capacity is measured in units of depth, usually centimeters or inches, and depends largely on the texture of the soil (Figure 10.10). Finer textures hold more water than coarser textures because fine particles have a much larger surface area in a unit of volume than coarse particles. So, a sandy soil has a small storage capacity, while a clay soil has a large storage capacity.
Figure 10.10 also shows the wilting point—the water storage level below which plants will wilt. It also depends on soil texture. Because fine particles hold water more tightly, it is more difficult for plants to extract moisture from fine soils. So plants can wilt in fine-textured soils even though they hold more soil water than coarse-textured soils. The difference between the storage capacity of a soil and its wilting point is the available water capacity—that is, the maximum amount of water available to plants when the soil is at storage capacity. The available water capacity is greatest in loamy soils.
SOIL-WATER BALANCE
It's clear that soil water is a critical resource needed for plant growth. The amount of water available at any given time is determined by the soil-water balance, which includes the gain and loss of water stored in the soil. Figure 10.11 is a diagram that illustrates the components of the soil-water balance.
Precipitation reaching the surface of the soil has two paths. One part enters the soil and recharges the reservoir of soil-water storage, while another part runs off the surface as overland flow into streams and rivers. Water leaves the soil-water storage reservoir through evapotranspiration, which depends on the air temperature and the amount of vegetation cover. Gravity also pulls water from the soil layer into ground water below. As we will see in a later chapter, the extra ground water eventually emerges from the bottoms of streambeds and lakes, joining overland flow to constitute total runoff.
During warm weather, evapotranspiration increases, and water flows out of the soil-water reservoir. If precipitation does not increase as well, soil water decreases. Eventually, soil-water storage can be drawn down below the wilting point, and plants and crops suffer. When precipitation increases or temperatures fall, reducing transpiration, the soil-water reservoir is recharged.
The soil-water balance is an important determiner of agricultural productivity, and it depends largely on climate—the variation in temperature and precipitation through the year. In a climate with a dry summer growing season, like the Mediterranean climate, irrigation is required for crops. In a climate with a wet summer or abundant year-round precipitation, such as the moist subtropical climate, soil water can maintain lush vegetation or crops through the growing season.